I started blogging
with the aim to write technical articles in the field of Aviation. Next to
opinion articles focused on readers with a certain background, my goal is to
write educational articles. These articles will be focused on Aviation
professionals, but with limited knowledge in other fields. I want to kick
these articles off with one of my favourite subjects within Aviation
Engineering: gas turbines, the power behind your flight.
Figure 1:
A JT9D high-bypass turbofan on the 'City of Everett', the first produced Boeing
747 prototype
How does an gas turbine work: the essentials
As the title suggests, a
simple way to explain the way a gas turbine works is ‘Suck, Squeeze, Bang,
Blow’. Air is sucked in, squeezed, ‘blown-up’ and blown away. But how is this
possible? Every gas turbine has three basis components: the compressor, the
combustor and the turbine. Figure 2
shows the interaction between these three components. Air flows from left to right.
Figure 2: Schematic view of a basic gas
turbine
Doing the unnatural: the compressor
The
compressor works with the same principle as a bicycle pump: air is rapidly
compressed. A bicycle tire is inflated up to pressures of around 6 bars, which
is six times the pressure around you. Small gas turbines have similar
compression ratios, but there is one difference: the compression of your tire
is reached with a lot of (exhausting) pumps, which could take a while. The
compression ratio of a gas turbine is reached within the blink of an eye and
with a lot more air to compress. Imagine pumping up your tire in a single
stroke, and that is for a small gas turbines. One of the biggest engines currently flying, the GE90 that powers the Boeing 777, has an airflow of about 1400 kg/s. That is sucking in the air of a 100 square meters big hangar every second! The newest developed gas turbines
have compression ratios reaching 60! To compare it with water pressure, this is
the water pressure at about 600 meters depth.
To achieve these high pressures, small
aerofoils ‘cooperate’ to create a high pressure. Figure 3
shows how an (axial) compressor works: rows of alternating spinning and fixed aerofoils
(rotors and stators) push the air through the narrowing compressor. The overall
effect is high pressure air. Because air naturally moves from high pressures to
low pressures (think about the daily weather forecast), the process within a
compressor is very unnatural. For this fact, compressor design is one of the
toughest parts within gas turbine design.
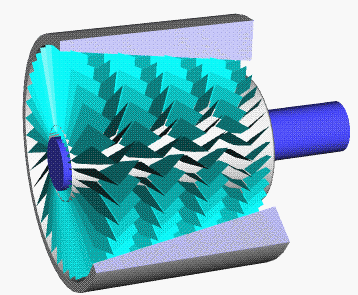
Figure 3: An (axial) compressor
The compressor driver: the turbine
The compressor needs a lot of
power to achieve the high compression ratio. This power is ‘harvested’ from the
turbine. The principle of a turbine is exactly the opposite of the compressor:
air moves from high pressure to a lower pressure and energy is used to drive
the shaft which powers the compressor. The design of a turbine also consist of
moving and static blades, but the blade design itself differ a bit (see Figure 4).
The difference is because of the difference in temperature: while the
compressor operates in temperature ranges of ambient temperature to 400/500
degrees Celsius, a turbine operates at temperatures around 1200/1300 degrees
Celsius (or higher!). To protect the metal blades from melting away, the blades are forged from exotic metals, cooled internally and externally and coated with a ceramic coating.
Figure 4: A compressor blade (left) versus a
turbine blade (right).
Where the magic happens: the combustor
A combination of a turbine and compressor could not operate
on itself. Because of inefficiencies, extra input of energy is required to keep
the compressor and turbine spinning. This is done by the combustion of fuel.
This combustion is done with a very high efficiency with the aim to minimize
the NOx, SOx and carbon monoxide (CO) emissions. The combustors of older gas turbines are less efficient which results in incomplete combustion (with by-products like soot and carbon monoxide), which clearly could be seen in Figure 6.
Figure 5: A Boeing 707 in take-off
Future goals
are set to repulse emissions by gas turbines and could be seen in Figure 5. The figure means that engines that are been developed by 2020 should emit 50% less carbon dioxide compared to engines developed in 2000.
Figure 6: ACARE flight path for Europe. Image
copyright of Arvind Gangoli Rao and Feijia Yin
Turbofan, Turbojet, Turbowhat?
Within (commercial) aviation, four ‘variants’ of gas turbine
exist: turbojets, turbofans, turboprops and turboshafts. These could be divided
in two categories: ‘using the power for thrust’ (turbojet & turbofan) and
‘using the power for torque’ (turboprop and turboshaft). Next to delivering
power for thrust or lift, gas turbines drive the electric, hydraulic and
pneumatic systems of aircraft.
In thrust we trust: the turbofan and turbojet
The turbofan (Figure
8)
and turbojet (Figure
7)
both rely on the same principle: generating ‘excess’ pressure in the exhaust to
generate thrust. You could say that a turbofan is a turbojet with a huge fan
mounted on front. The fan, because of the bypass, is more efficient than the
turbojet and therefore is applied widely in the civil industry. Modern day
turbofans are designed with bigger bypasses to enhance the efficiency.
Figure 7: a turbojet schematic and the
General Electric J85-GE-17A
For military application and in particular for fighter jets,
turbofans with high bypass ratios are not useful. For most cases, a turbojet or
a turbofan with a low bypass ratio is used. To enhance performance in some
scenarios, an afterburner is used. An afterburner is basically a huge combustor
mounted within the exhaust, so extra energy is added. With the right use of the
exhaust, velocities above the speed of sound could be reached.
Figure 8: A turbofan schematic (left) and the
CFM56-2
Small, but powerful: the turboshaft and turboprop
The principle of the turboprop (Figure 9)
and turboshaft (Figure
10)
is not to generate thrust, but to convert all the power that is generated into
torque. The gas turbine is used as a ‘hot gas generator’. The energy from that
gas is then extracted mostly by a power turbine: an extra turbine which is not
coupled to a compressor but to a gearbox which powers a propeller or
(helicopter) rotor.
Figure 9: Turboprop schematic and the
T56-15-LFE
The difference between a turboprop and turboshaft is that mostly one turboprop powers one propeller, but a helicopter rotor could be powered by more than one turboshaft. For instance, the AH-64 ‘Apache’ helicopter has two GE T700 turboshafts, which are both able to power the helicopter by itself. The extra gas turbine is installed to provide (extra) power for certain manoeuvres and for redundancy.
Figure 10: Turboshaft schematic and the
T55-GA-714A
What’s else?
Gas Turbines are used for more purposes than described
before. The same principle as turboprops and turboshaft could be used to drive
anything other than a propeller or rotor. An Auxiliary Power Unit (APU) is a
‘mini’ gas turbine installed in the tail of most (civil) aircraft. The APU
provides power for hydraulic, pneumatic and electric systems if the engines are
not started yet. The high-pitched sound that could be heard at airports is
coming from the APU. It could also be used if both engines fail in mid-flight
to power the essential systems to keep the aircraft in control. A deviation
from the turboprop is applied in the maritime field. For instance, Areal
Commando Frigates use gas turbines for high speeds. Gas turbines are also used
to generate high power current. Special gas turbines are designed for energy
generation purposes and are enhanced with intercoolers, heat exchangers and are
not limited in size. Most of the times a combined cycle is used: steam that is
generated with exhaust gasses powers one or more steam turbines. This enhances
the total efficiency of the power plant.
Figure 11:
Three other major applications of gas turbines: the APU, maritime propulsion
and energy generation
What’s next?
Because of environmental boundaries and the depletion of the
oil reserves, within this century the aviation industry has to find other means of propulsion. This could be done by using other fuels, going hybrid or even
fully electric. I will come back on this subject next month in a separate
article on the short- and long term alternatives for gas turbines.
Figure 12: What's next? Image copyright of NASA
I hope I taught you a thing or two about the way gas turbines work. If any questions arise do not hesitate to comment below! Next two blogs I will
focus on the ILA Berlin Air Show which I will visit on April 27th.
In two separate articles I will preview and review the air show, the aircraft
and technologies displayed at the trade visitors’ event.
No comments:
Post a Comment